Scientists have for the first time documented areas in the deep earth where materials have undergone changes on a subatomic level. There, crushing pressures apparently are bringing about a long hypothesized but until now unproven quantum phase transition called a spin crossover, which affects the magnetic state of a key deep-earth mineral.
The interior of the earth is a mystery, especially at depths greater than about 650 kilometers, or some 400 miles. Researchers have only seismic tomographic images of this region; to interpret them, they need to measure or calculate the velocities of seismic waves passing through minerals in various layers. Direct measurements are challenging and rare but in the last two decades scientists have gotten around this to some degree by making quantum calculations. With these, they can create 3D maps and figure out the mineralogy and temperature of the observed regions. When a phase transition occurs in a mineral, such as a crystal structure change, scientists observe a velocity change, usually a sharp seismic velocity discontinuity.
In 2003, lab scientists observed a novel type of phase change in minerals—a spin change in iron in ferropericlase, the second most abundant component of the earth’s lower mantle. A spin change, or spin crossover, can happen in minerals like ferropericlase under an external stimulus, such as pressure or temperature. Over the next few years, experimental and theoretical groups confirmed this phase change in both ferropericlase and bridgmanite, the most abundant phase of the lower mantle. But no one was quite sure why or where this was happening.
Columbia Engineering professor Renata Wentzcovitch began investigating this question. Wenzcovitch’s research focuses on computational quantum mechanical studies of materials at extreme conditions, in particular planetary materials. In 2006, she published her first paper on ferropericlase, providing a theory for the spin crossover. Her theory suggested it happened across a thousand kilometers in the lower mantle.
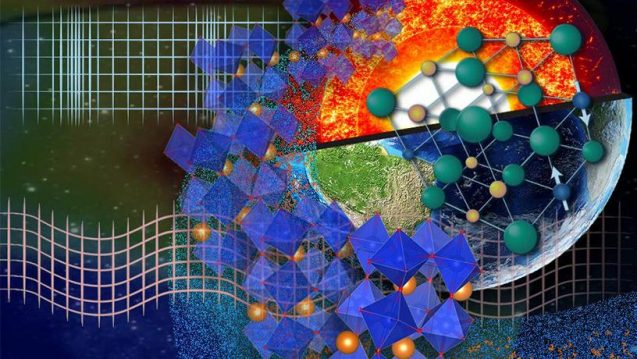
Since then, Wentzcovitch, a professor in Columbia’s department of Applied Physics and Applied Mathematics, and the university’s Lamont-Doherty Earth Observatory, has published 13 papers with her group on the topic. In these, they investigated velocities in every possible situation of the spin crossover in ferropericlase and bridgmanite, and predicted properties of these minerals throughout this crossover. In 2014, they predicted how this spin change could be detected in seismic tomographic images, but seismologists still could not see it.
With a multidisciplinary team from the University of Oslo, the Tokyo Institute of Technology and Intel Co., Wenzcovitch’s latest paper, in the journal Nature, details how they have now identified the ferropericlase spin crossover signal deep within the earth’s lower mantle. They achieved this by looking at specific regions in the mantle where ferropericlase is expected to be abundant.
“This exciting finding, which confirms my earlier predictions, illustrates the importance of materials physicists and geophysicists working together to learn more about what’s going on deep within the earth and other planets,” said Wentzcovitch. “Geodynamic simulations have shown that the spin crossover invigorates convection in the earth’s mantle and tectonic plate motion. So we think that this quantum phenomenon also increases the frequency of tectonic events such as earthquakes and volcanic eruptions.”
Engineers commonly harness spin transition in materials like those used for magnetic recording. If you stretch or compress just a few nanometer-thick layers of a magnetic material, you can change the layer’s magnetic properties and improve the medium’s recording properties. Wentzcovitch’s new study shows that the same phenomenon happens across thousands of kilometers in the planet’s interior, taking this from the nano- to the macro-scale.
“There are still many regions of the mantle researchers do not understand, and [studying] spin state change is critical to further progress,” said Wentzcovitch. She is continuing to interpret seismic tomographic maps, and developing more accurate materials simulation techniques to predict seismic velocities and transport properties. In particular, she is looking at regions rich in molten or near-molten iron. “We’ll be able to improve our analyses of 3D tomographic images of the earth and learn more about how the crushing pressures of the [interior] are indirectly affecting our lives above,” she said.